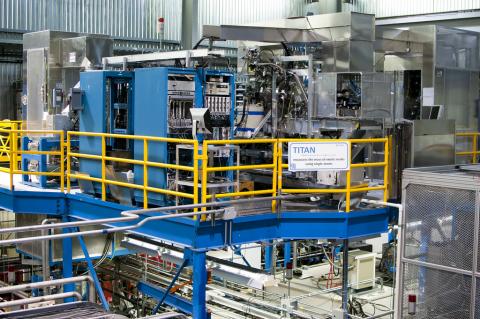
In the middle of the last century when people were trying to understand the basic properties of atoms, mass measurements were used to look into the inner workings of objects. The mass, as we know, is linked to the energy (in this case, the binding energy needed to hold an atom together), via Einstein’s famous ‘E=mc2’ and hence allows one to detect extra stable or more stable atoms (or nuclei). These measurements eventually led to the development of the Nuclear Shell Model in analogy to the Atomic Shell Model. In 1963, the Nobel Prize in Physics was awarded to Maria Goeppert Mayer, J. Hans D. Jensen for their discovery. They had found that specific combinations of neutrons and protons, the constituents of nuclei (or atoms, when adding the electrons), had extra stability in their binding energy. These combinations were labelled Magic Numbers. The Shell Model that they developed could explain those Magic Numbers, however only for a certain, albeit large, subset of all known nuclei.
Precise and accurate measurements of the mass of the neutron-rich calcium and potassium isotopes 51,52Ca and 51K have recently been carried out for the first time at TRIUMF's Ion Trap for Atomic and Nuclear Science (TITAN) facility. The measured masses deviate greatly from what was previously known and help to shed light on the evolution of nuclear forces in neutron-rich nuclei, providing important guides for new theoretical predictions.
Calcium, an important element in biological systems, is also of great interest in nuclear physics. The most abundant isotope, 40Ca, which has 20 protons and 20 neutrons, is the heaviest stable element that has an equal number of protons and neutrons. Another peculiar feature of calcium isotopes is that it is the only element that contains two (nearly stable) doubly-"magic" nuclei: calcium-40 and calcium-48. 40Ca is stable, while the half-life of 48Ca is over a billion times longer than the age of the universe and hence can be considered stable as well. A magic number in nuclear physics occurs when a certain number of nucleons (either protons or neutrons) arrange themselves into shells, much like atomic orbitals for electrons. The nuclear established magic numbers are 2, 8, 20, 28, 50, 82, and 126.
Recently, physicists have been trying to develop theories based on quantum chromodynamics (or QCD, the fundamental theory of the strong force) to predict elements of nuclear physics. An important feature that came out of these attempts to link nuclear theory and QCD was the possibility to calculate so-called "three-body" forces. Three-body forces occur when three nucleons interact simultaneously. In previous theories, it was assumed that nucleons would only interact with each other one at a time. This was a reasonable approach because the computer power required to calculate three-body forces were developed in the last decade. Three-body forces have also begun to explain changes in the well-known magic numbers far from stability. It has been found that ‘new’ magic number can arise, and the well-known magic numbers can disappear or migrate! This is important in the calcium isotopes, as new "sub-magic" neutron numbers of 32 and 34 have been predicted to appear, and are awaiting experimental conformation ("sub-magic" nuclei are more stable than their neighbours, but not as stable as magic nuclei). The rise of three-body theories also allow for parameter free predictions, i.e. these theories do not need to be "adjusted" to agree with experiment. With this improved version of the nuclear force, it was possible for theorists to calculate properties of the neutron rich calcium isotopes and compare them to the mass measurements from TITAN.
The result? The agreement between experiment and theory is remarkable and is shown in the figure above. While older phenomenological theories (green lines) also agree very well with the new experimental values, it is important to remember that they have had their theories tweaked using inputs from nearby nuclei in order to give accurate predictions. The three-body (blue lines) have not had the same tweaking, and are equally as good at predicting the observed trend. Interestingly, the TITAN experiments, have spurred significant theoretical activities, and a second theory paper has been published in the same issue of Physical Review Letters (G. Hagen et al.), where they find remarkable agreement, albeit using a different theoretical framework, but also employing three-body forces. The large observed shifts, if they also exist in heavier nuclei, and the prediction of the abundances of nuclei heavier than iron, may be important for nuclear astrophysics. This experimental conformation of three-body forces in medium mass nuclei is also important for predictions of neutron star matter, as the three-body forces are the same in nuclear and neutron star matter.
The paper describing this work has been published by Physical Review Letters.
-- by Aaron T. Gallant (Ph.D. student UBC, recipient of the A.G. Bell Scholarship)